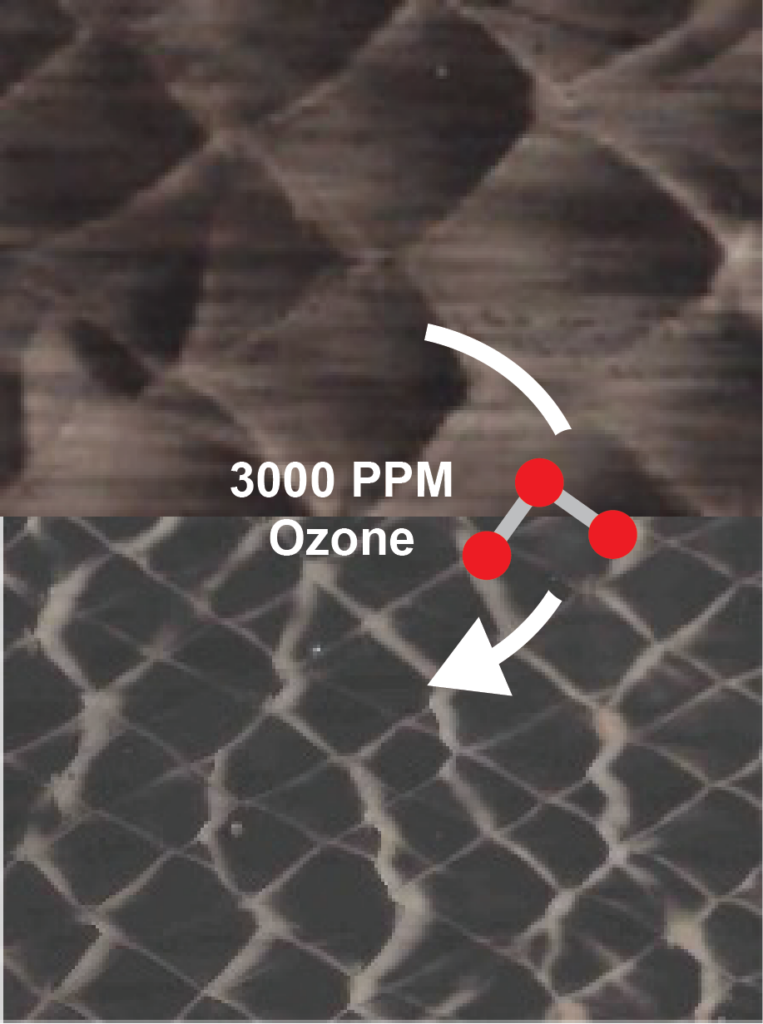
Controlling detonation instabilities
Detonation engines have the potential to dramatically improve propulsion and stationary power generation efficiency, translating to reduced emissions in these sectors. Development is hindered, however, by severe detonation instabilities in complex and constrained engine conditions. Our past work showed it is possible to control these instabilities using dopant quantities of additives, like ozone. Discovering approaches to modulate detonation instability directly realizable in production engines is one of our current research aims.
Rapid modeling of detonation in complex geometries
Detonation engine development, detonation suppression, and detonation-based material synthesis all require accurate models to predict propagation. These applications rely either on empirical correlations which have limited applicability or on CFD simulations which are extremely expensive and difficult to perform. We pioneered a new approach that can run on a laptop: a model that simulates macroscopic detonation propagation by leveraging detonation physics and pre-calculated blast dynamics. We are further improving the model to be more versatile and expanding the methodology to perform 3D simulations.
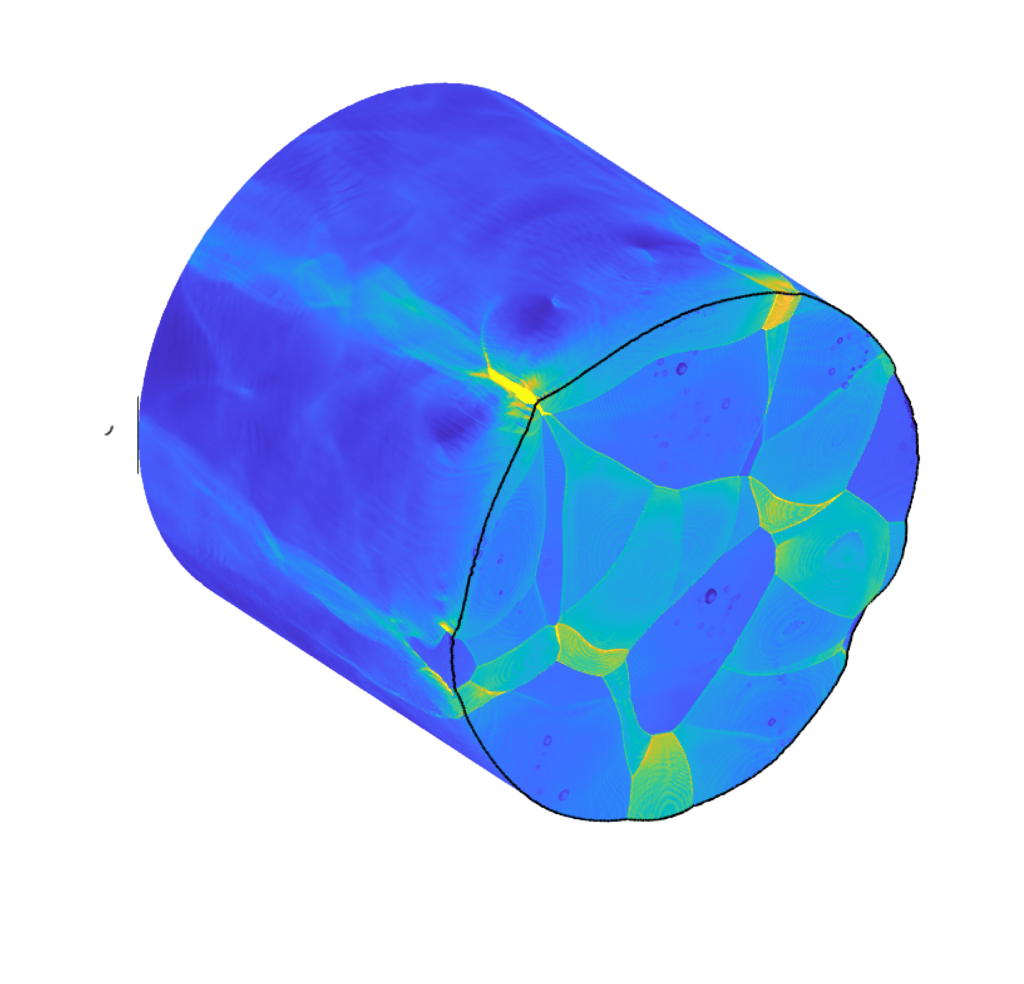
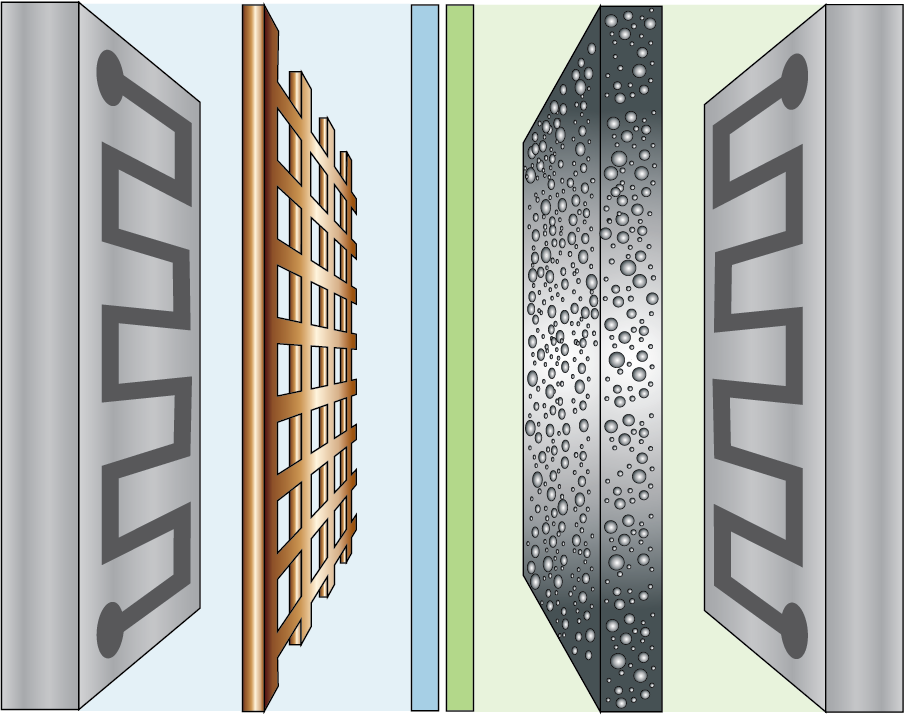
Modeling and optimization of fuel synthesis via electrocatalysis
Synthesizing renewable fuels via CO2-reduction electrocatalysis offers a pathway to decarbonize sectors that are reliant on liquid fuels such as aviation and remote power generation. To better design electrochemical devices, the transport and chemical environment must be well-understood and controlled. We are building multiphysics models to better understand and optimize the dynamics within these devices. We are also active in optimizing the fuel performance resultant from electrochemical synthesis.